Introduction
Agriculture accounts for approximately 23% of global greenhouse gas emissions while simultaneously offering one of the most scalable opportunities for carbon sequestration and climate change mitigation. Carbon farming—the implementation of agricultural practices that remove carbon dioxide from the atmosphere and store it in soil organic matter and perennial vegetation—represents a promising approach to transform agricultural systems from net emitters to net sinks of greenhouse gases. Beyond climate benefits, carbon farming practices enhance soil health, water retention, biodiversity, and farm resilience, creating multiple win-win scenarios for farmers and ecosystems. This article examines the scientific foundations of carbon farming, evaluates specific management practices and their effectiveness, addresses implementation challenges and economic considerations, and explores pathways toward broader adoption of these climate-smart agricultural approaches.
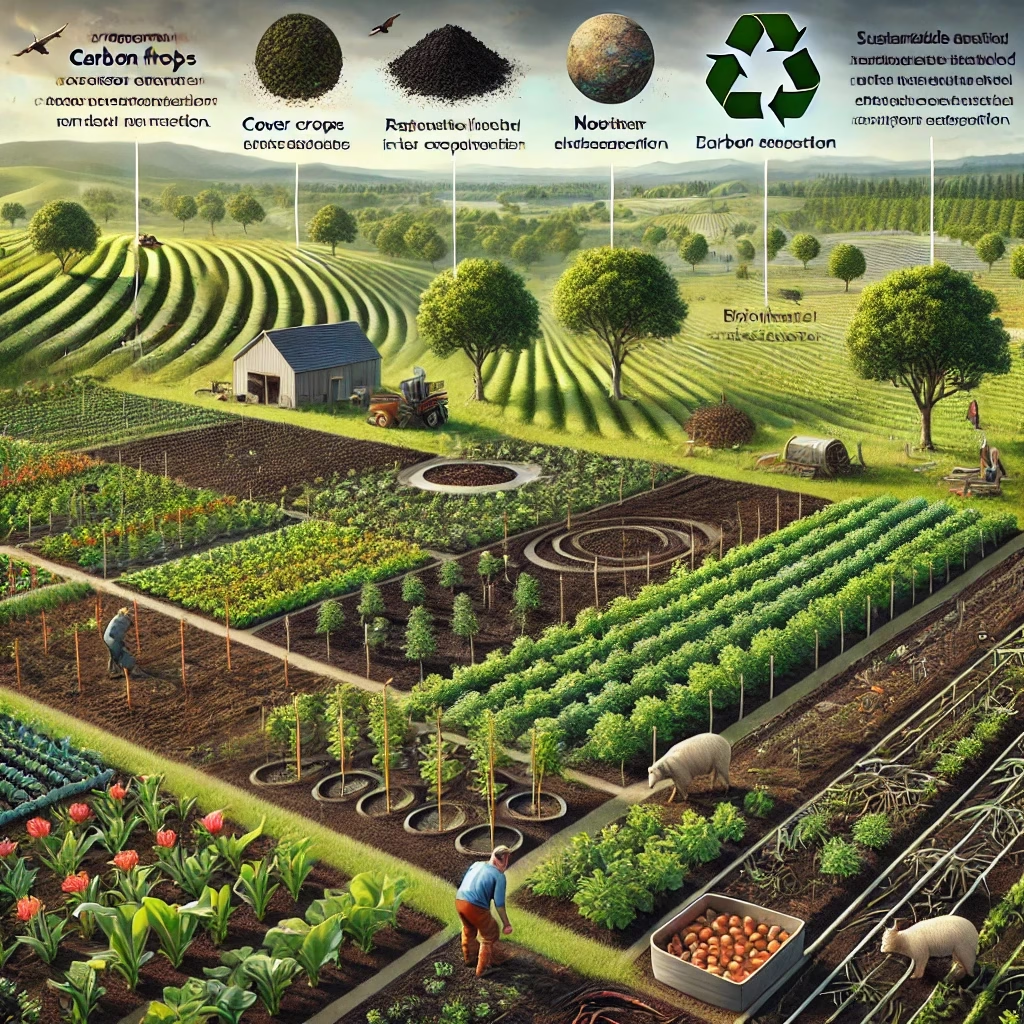
The Science of Agricultural Carbon Sequestration
Agricultural ecosystems store carbon through several interconnected biological processes that can be enhanced through appropriate management:
- Photosynthetic Carbon Capture
The foundation of carbon sequestration begins with photosynthesis, where plants convert atmospheric CO₂ into carbohydrates. Agricultural systems with extended photosynthetically active periods capture more carbon. Research demonstrates that extending active growth periods by 30-60 days annually through cover cropping or perennialization can increase carbon fixation by 1-3 metric tons CO₂-equivalent (CO₂e) per hectare per year. Studies in California vineyards show that maintaining cover crops between rows increases annual carbon fixation by 0.8-1.2 metric tons CO₂e per hectare compared to bare soil management. - Carbon Allocation Patterns
Plants allocate carbon to various tissues, with substantial differences in persistence and sequestration potential:- Above-ground biomass: Carbon in annual crops typically returns to the atmosphere within 1-2 years unless biomass is incorporated into more stable forms. Conversely, perennial systems accumulate carbon in woody tissues, with studies showing that agroforestry systems sequester 0.5-4.0 metric tons CO₂e per hectare annually in above-ground biomass depending on species composition and climate.
- Below-ground biomass: Root systems contribute significantly to soil carbon. Deep-rooted perennials allocate 40-60% of photosynthates below ground compared to 20-30% in annual crops. Research demonstrates that switchgrass can develop root systems containing 5-12 metric tons of carbon per hectare within 3-5 years of establishment.
- Root exudates: Plants release 15-40% of fixed carbon as exudates that feed soil microorganisms and contribute to stable soil organic matter. Studies using carbon isotope tracing show that root exudates can contribute 30-50% more to stable soil carbon pools than above-ground residues.
- Microbial Processing and Carbon Stabilization
Soil microorganisms play crucial roles in transforming plant-derived carbon into stable forms:- Microbial carbon pump: The conversion of plant compounds through microbial metabolism produces microbial necromass and metabolites that persist longer than original plant materials. Research indicates that 50-80% of stable soil carbon derives from microbial processing rather than direct plant inputs.
- Aggregation processes: Bacterial and fungal activity creates soil aggregates that physically protect carbon from decomposition. Studies show that well-aggregated soils can protect 20-40% more carbon than structurally degraded soils with equivalent carbon inputs.
- Mineral associations: Carbon molecules bind to soil minerals, particularly clay particles, creating complexes that resist decomposition for decades to centuries. Research demonstrates that soils with higher clay content can stabilize 2-5 times more carbon than sandy soils under equivalent management.
- Greenhouse Gas Dynamics
Carbon farming requires consideration of all greenhouse gases, not just CO₂:- Nitrous oxide (N₂O): With 265-298 times the global warming potential of CO₂, N₂O emissions from nitrogen fertilizers can offset carbon gains. Studies show that precision nitrogen management can reduce N₂O emissions by 30-50% compared to conventional practices.
- Methane (CH₄): With 28-36 times the warming potential of CO₂, methane emissions from livestock and flooded rice fields significantly impact the greenhouse gas balance. Research indicates that feed additives can reduce enteric methane from ruminants by 20-40%, while alternate wetting and drying in rice cultivation can reduce methane emissions by 30-70%.
- Net greenhouse gas balance: Successful carbon farming achieves a negative greenhouse gas balance when sequestration exceeds emissions. Meta-analyses indicate that integrated systems combining multiple carbon-positive practices typically achieve net negative emissions of 2-7.5 metric tons CO₂e per hectare annually.
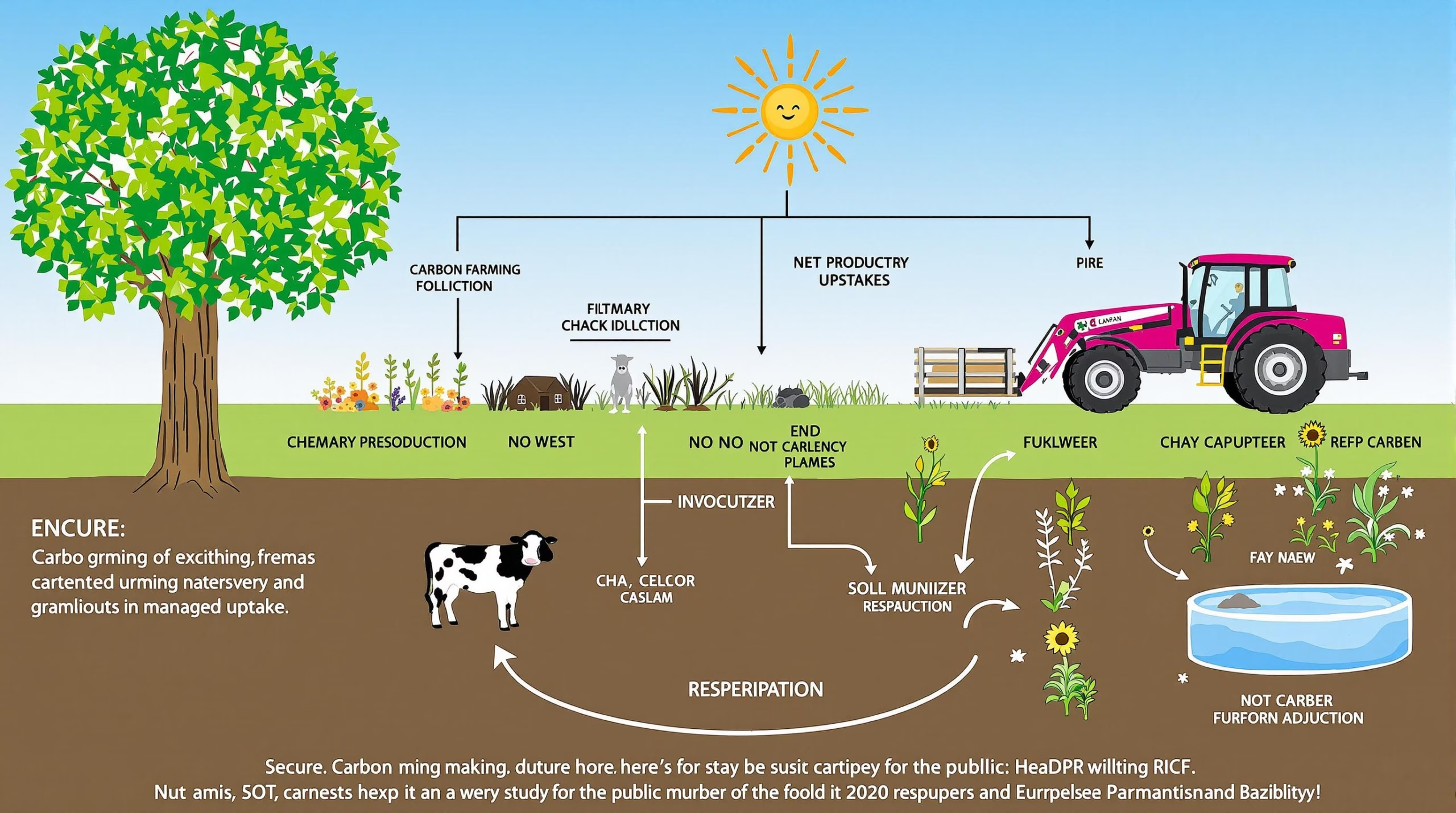
Evidence-Based Carbon Farming Practices
Multiple agricultural practices demonstrate the potential to enhance carbon sequestration while providing additional ecosystem benefits:
- Cropland Soil Management
Practices that minimize soil disturbance and maximize carbon inputs show significant sequestration potential:- Reduced or no-tillage: Minimizing soil disturbance preserves soil structure and protects existing carbon stocks. Meta-analyses indicate average sequestration rates of 0.3-0.6 metric tons CO₂e per hectare annually for the first 20 years after conversion to no-till, with higher rates (0.5-0.9 metric tons) in humid climates and lower rates (0.1-0.3 metric tons) in arid regions.
- Cover cropping: Growing cover crops during fallow periods increases carbon inputs and extends photosynthetic activity. Research demonstrates sequestration rates of 0.4-1.2 metric tons CO₂e per hectare annually, with legume-grass mixtures typically achieving higher rates than single-species covers due to greater biomass production and nitrogen-carbon synergies.
- Crop rotation diversification: Diverse rotations with varying root architectures explore different soil niches and enhance microbial diversity. Studies show that adding one additional crop to simple rotations increases carbon sequestration by 0.2-0.4 metric tons CO₂e per hectare annually, with further additions showing diminishing but positive returns.
- Organic amendments: Compost, manure, and biochar provide recalcitrant carbon directly while stimulating additional plant growth. Research on compost application shows typical sequestration rates of 0.7-3.0 metric tons CO₂e per hectare annually depending on application rates, with particularly high efficiency in degraded soils.
- Perennial Integration
Incorporating perennial plants into agricultural landscapes significantly enhances carbon sequestration:- Agroforestry systems: Integrating trees with crops or livestock creates multi-strata carbon capture. Meta-analyses show sequestration rates of 1.0-6.3 metric tons CO₂e per hectare annually in above and below-ground biomass plus soil carbon, with higher rates in tropical systems (3.0-6.3 metric tons) compared to temperate regions (1.0-3.3 metric tons).
- Perennial grains and crops: Developing and adopting perennial alternatives to annual staples provides continuous living cover. Research on Kernza® (intermediate wheatgrass) indicates soil carbon accrual of 0.8-1.7 metric tons CO₂e per hectare annually compared to annual wheat systems, with parallel studies on perennial rice showing similar advantages.
- Silvopasture: Integrating trees into livestock grazing systems creates three-dimensional carbon capture while providing animal welfare benefits. Studies document sequestration rates of 1.0-4.0 metric tons CO₂e per hectare annually, with additional methane mitigation benefits when trees provide condensed tannins that reduce enteric fermentation.
- Orchard and vineyard floor management: Maintaining living ground covers in perennial cropping systems enhances total system carbon. Research demonstrates that transitioning from clean cultivation to permanent cover crops in orchards increases carbon sequestration by 0.5-1.0 metric tons CO₂e per hectare annually while improving water infiltration and reducing erosion.
- Grassland and Rangeland Management
Practices that enhance grassland productivity and carbon allocation show significant potential:- Managed grazing systems: Appropriate grazing timing, intensity, and recovery periods enhance plant productivity and root development. Research on adaptive multi-paddock grazing shows net sequestration rates of 0.5-3.0 metric tons CO₂e per hectare annually compared to continuous grazing, with higher rates during ecosystem recovery phases and lower rates as systems approach new equilibria.
- Grassland restoration: Reestablishing perennial grasslands on degraded lands creates significant carbon sinks. Studies demonstrate sequestration rates of 1.0-3.0 metric tons CO₂e per hectare annually for the first 10-20 years after restoration, with rates gradually declining as new steady states are reached.
- Species composition management: Introducing deep-rooted species and optimizing plant diversity enhances carbon allocation below ground. Research shows that increasing native perennial grass representation by 10-20% in degraded rangelands can increase carbon sequestration by 0.3-0.7 metric tons CO₂e per hectare annually.
- Fire management: Implementing prescribed burning regimes that mimic historical fire patterns can enhance grassland health and belowground carbon. Studies indicate that appropriate fire intervals increase root-to-shoot ratios by 15-40% and enhance mycorrhizal associations, creating more persistent carbon pools.
- Rice Cultivation Systems
Modified rice production practices can dramatically reduce methane emissions while maintaining or enhancing yields:- Alternate wetting and drying (AWD): Periodically allowing rice fields to drain instead of continuous flooding reduces anaerobic methanogenesis. Research documents 30-70% reductions in methane emissions, equivalent to avoiding 1.0-4.0 metric tons CO₂e per hectare annually, with minimal yield impacts when properly implemented.
- Mid-season drainage: Brief drainage periods during the growing season reduce methane production while minimizing yield penalties. Studies show emission reductions of 15-40% compared to continuous flooding, equivalent to avoiding 0.5-2.0 metric tons CO₂e per hectare annually.
- Rice straw management: Avoiding off-season flooding when incorporating rice straw reduces methane production. Research demonstrates that aerobic decomposition of straw reduces methane emissions by 60-90% compared to anaerobic decomposition in flooded conditions.
- Improved rice varieties: Selecting cultivars with higher yields, lower methane emissions, or both improves the greenhouse gas intensity of production. Studies show variations of 20-40% in methane emissions among different varieties under identical management.
- Integrated Systems Approaches
Combining multiple carbon-friendly practices creates synergistic benefits:- Conservation agriculture: Integrating minimum tillage, permanent soil cover, and crop rotation creates multiple carbon-enhancing mechanisms. Meta-analyses show that full conservation agriculture systems sequester 0.5-1.7 metric tons CO₂e per hectare annually more than partial implementations.
- Integrated crop-livestock systems: Cycling nutrients between crops and animals while incorporating perennials enhances system efficiency. Research demonstrates net sequestration of 1.0-3.5 metric tons CO₂e per hectare annually in well-designed systems, with additional benefits from reduced external input requirements.
- Regenerative agriculture: Holistic approaches emphasizing soil health, biodiversity, and ecosystem function show promising results. Studies of farms using comprehensive regenerative approaches document net sequestration of 2.0-7.5 metric tons CO₂e per hectare annually during transition phases, stabilizing at lower but positive rates in later years.
Economic Considerations and Implementation Pathways
Translating carbon farming potential into practical reality requires addressing economic barriers and creating enabling conditions:
- Economic Barriers and Opportunities
Carbon farming practices present both costs and benefits that influence adoption decisions:- Implementation costs: Transition costs for carbon farming practices range from $20-500 per hectare, with higher costs typically associated with perennial establishment and infrastructure development. Studies show that no-till conversion costs $50-150 per hectare initially but reduces operational costs by $30-80 per hectare annually thereafter.
- Risk and uncertainty: Many practices require skills development and involve yield uncertainty during transition periods. Research indicates that farmer networks and staged implementation approaches can reduce perceived risk by 40-60% and improve success rates by 30-50%.
- Time lags: Carbon accumulation occurs gradually while costs are often front-loaded. Economic analyses show that without incentive payments, breakeven periods range from 2-3 years for some soil management practices to 5-15 years for agroforestry systems, creating adoption barriers despite positive long-term returns.
- Co-benefits: Many carbon farming practices deliver valuable ecosystem services beyond carbon sequestration. Research demonstrates that improved water infiltration alone provides $30-250 per hectare in annual value through reduced irrigation needs or flood mitigation, while enhanced biodiversity can reduce pest control costs by $20-100 per hectare annually.
- Carbon Markets and Payment Mechanisms
Emerging carbon markets offer potential revenue streams for farmers adopting carbon-sequestering practices:- Voluntary carbon markets: Private markets trading carbon credits from agricultural projects have grown by 40-70% annually in recent years. Analysis shows price ranges of $5-50 per metric ton CO₂e, with higher prices typically associated with projects offering verified co-benefits.
- Compliance markets: Government-regulated carbon markets increasingly include agricultural offsets. Research indicates that stable compliance markets typically offer 20-60% price premiums compared to voluntary markets but involve higher verification requirements costing $5-15 per hectare annually.
- Supply chain initiatives: Major food companies are developing carbon insetting programs within their supply chains. Industry data shows premium payments of 5-20% for carbon-beneficial products, potentially offering more reliable returns than external carbon markets.
- Ecosystem service stacking: Combining carbon payments with compensation for water quality, biodiversity, and other ecosystem services enhances economic viability. Research demonstrates that stacked incentive programs can increase farmer returns by 40-120% compared to carbon-only payments.
- Monitoring, Reporting, and Verification Challenges
Credible carbon accounting requires robust measurement systems:- Direct measurement approaches: Physical soil sampling remains the gold standard but is costly at $200-600 per farm for comprehensive assessment. Research shows that stratified sampling protocols can reduce costs by 40-60% while maintaining 85-95% of the accuracy of more intensive protocols.
- Model-based approaches: Process models combined with key measurements offer cost-effective verification. Analysis indicates that well-calibrated models achieve 70-90% of the accuracy of intensive measurement protocols at 10-20% of the cost.
- Remote sensing integration: Satellite and drone data increasingly support carbon monitoring. Studies demonstrate that combining remote sensing with strategic ground truthing can reduce monitoring costs by 50-70% in extensive systems while improving spatial resolution.
- Practice-based protocols: Some programs pay for implementing specific practices rather than directly measuring carbon outcomes. Research shows that practice-based approaches achieve 60-80% of the precision of measurement-based approaches at 20-30% of the verification cost.
- Policy and Institutional Support
Enabling policies can dramatically accelerate carbon farming adoption:- Direct incentive programs: Government payment programs supporting carbon-beneficial practices show adoption rates 3-5 times higher than market-only approaches. Analysis of the Conservation Reserve Program in the United States demonstrates sequestration of approximately 7 million metric tons CO₂e annually at costs of $25-100 per metric ton.
- Research and extension: Public investment in region-specific carbon farming research and knowledge transfer shows high returns. Studies indicate that each dollar invested in carbon farming extension typically yields $5-15 in implemented practices and subsequent carbon sequestration.
- Risk reduction mechanisms: Insurance programs and transition payments that buffer uncertainty accelerate adoption. Research shows that reducing downside risk by 50% through targeted programs can increase practice adoption by 30-90% depending on the practice and context.
- Long-term land tenure: Secure land tenure significantly influences willingness to invest in carbon farming. Data indicates that farmers with secure tenure are 2-4 times more likely to implement practices with delayed returns compared to those with short-term arrangements.
Case Studies of Carbon Farming Success
Examining successful implementations provides insights into effective approaches across different agricultural contexts:
- Marin Carbon Project (California, USA)
This pioneer initiative demonstrated the potential of compost application on rangelands. A single application of 1/2 inch of compost increased forage production by 40-70% and soil carbon sequestration by 0.4-1.0 metric tons CO₂e per hectare annually, with effects persisting more than 10 years after application. Cost-benefit analysis showed that increased forage value alone covered compost costs within 3-6 years, while carbon benefits provided additional value. The project has expanded to over 100 ranches implementing carbon plans across 40,000+ hectares. - Carbon Farmers of Australia
This program supports Australian farmers implementing carbon farming across vast rangeland areas. Participating properties implementing rotational grazing and vegetation management have documented average sequestration rates of 0.5-2.0 metric tons CO₂e per hectare annually across millions of hectares. Financial returns include carbon credits valued at AU$15-35 per metric ton CO₂e plus production benefits from improved grazing land condition. The program demonstrates successful linkage between national carbon policy, scientific measurement, and practical farm implementation. - 4 per 1000 Initiative Demonstration Farms (France)
France’s national “4 per 1000” initiative promotes practices to increase soil carbon by 0.4% annually. Demonstration farms implementing cover crops, reduced tillage, agroforestry, and organic amendments have achieved average sequestration rates of 0.8-1.5 metric tons CO₂e per hectare annually. Economic analysis shows variable financial outcomes depending on specific practices, with cover cropping and reduced tillage typically breaking even within 3-5 years through input reductions, while agroforestry systems required 8-12 years to reach profitability despite higher long-term returns. - COMACO Landscape Management Project (Zambia)
This integrated initiative in Zambia’s Luangwa Valley works with 200,000+ smallholder farmers implementing conservation agriculture, agroforestry, and improved cookstoves. Carbon sequestration rates average 2.0-4.5 metric tons CO₂e per hectare annually during the first 10 years, with higher rates in agroforestry components. Program success derives from linking carbon-beneficial practices to premium markets for farmer products, creating immediate economic incentives beyond carbon value. Participating farms have increased yields by 30-150% while reducing deforestation by 60-70%. - The Land Institute’s Perennial Grain Development (Global)
This research program is developing perennial grain crops to replace annual counterparts. Field trials with Kernza® perennial wheat show soil carbon increases of 0.8-1.7 metric tons CO₂e per hectare annually compared to annual wheat systems, plus 15-30% reductions in nitrogen leaching and 50-90% reductions in sediment loss. Economic analysis indicates that despite 30-50% lower grain yields than annual wheat, Kernza becomes economically competitive through eliminated replanting costs, reduced input requirements, and emerging premium markets for climate-beneficial grains.
Future Directions and Research Needs
Several emerging areas promise to enhance carbon farming effectiveness and adoption:
- Advanced Carbon Sequestration Practices
Research is exploring practices with exceptionally high sequestration potential:- Deep soil carbon management: Techniques targeting carbon placement at 30-100+ cm depths, where decomposition rates are 5-10 times slower than surface soils. Early research on deep-rooted cover crops and deep soil inversion shows potential sequestration rates 2-3 times higher than conventional approaches.
- Mineral weathering enhancement: Accelerating natural weathering processes by applying crushed silicate rocks to agricultural soils. Research indicates sequestration potential of 0.3-1.2 metric tons CO₂e per hectare annually plus soil fertility benefits, though questions remain about lifecycle energy requirements.
- Biochar systems: Pyrolyzing biomass into stable carbon forms that persist for centuries in soil. Meta-analyses show average soil carbon increases of 25-45% with biochar application, with residence times of 100-1000+ years depending on production conditions and soil environment.
- Blue carbon agriculture: Expanding carbon farming into coastal environments through seaweed cultivation and wetland restoration. Research demonstrates sequestration rates of 5-25 metric tons CO₂e per hectare annually in these systems, far exceeding typical terrestrial rates.
- Technological Innovations
Emerging technologies are improving implementation efficiency and verification:- Carbon farming decision support systems: AI-powered tools integrating soil, climate, and management data to optimize carbon sequestration strategies. Early implementations show 30-50% improvements in practice selection and placement compared to generalized recommendations.
- Precision conservation technologies: GPS-guided equipment for precise implementation of practices such as contour plantings and organic amendment application. Research demonstrates 15-30% improvements in carbon outcomes through optimal spatial application.
- Low-cost soil carbon sensing: Spectroscopic and other rapid assessment methods reducing measurement costs by 70-90% compared to traditional laboratory analysis. Validation studies show accuracy of 80-95% relative to conventional methods when properly calibrated.
- Blockchain and digital verification: Distributed ledger technologies creating transparent carbon accounting systems. Pilot projects indicate 40-60% reductions in verification costs and 50-75% faster credit issuance compared to traditional verification approaches.
- Genetic Resources and Plant Breeding
Developing crop varieties specifically designed for carbon sequestration:- Root architecture optimization: Breeding crops with deeper, more extensive root systems that place carbon deeper in the soil profile. Research shows 20-50% variations in root biomass allocation among cultivars of the same species, offering significant selection potential.
- Enhanced rhizodeposition: Selecting for varieties with greater carbon exudation that feeds soil microbial communities. Early research indicates 15-35% variation in rhizodeposition rates among cultivars, correlating with differences in soil carbon accumulation.
- Perennialization breeding: Developing perennial versions of currently annual staple crops through domestication of wild perennials or hybridization. Active breeding programs exist for perennial wheat, rice, sorghum, and oilseeds, with early field trials showing 40-80% of annual crop yields plus significant carbon benefits.
- Microbiome engineering: Selecting plant varieties that recruit beneficial soil microbes enhancing carbon sequestration. Research demonstrates that host genetics can influence rhizosphere bacterial and fungal communities by 10-30%, affecting carbon cycling processes.
- Social and Behavioral Research
Understanding and addressing human dimensions of carbon farming adoption:- Farmer decision processes: Research on how farmers evaluate carbon farming opportunities and make implementation decisions. Studies show that peer networks influence adoption 3-5 times more strongly than technical information alone, highlighting the importance of social learning.
- Behavioral economics applications: Using insights from behavioral science to design more effective incentive programs. Research demonstrates that upfront payments with clawback provisions increase adoption by 40-60% compared to equivalent payments provided retrospectively.
- Cultural and indigenous knowledge integration: Incorporating traditional ecological knowledge into carbon farming approaches. Studies show that indigenous management systems often sequester 30-50% more carbon than conventional approaches in comparable environments, offering important insights for broader application.
- Land tenure innovations: Developing models that align carbon incentives with land tenure realities. Research on long-term carbon covenant programs shows 2-3 times higher adoption rates compared to contract-based approaches of equivalent value but shorter duration.
- Policy and Market Development
Creating enabling conditions for scaled carbon farming implementation:- Integrated policy frameworks: Developing coherent policies across climate, agriculture, environment, and trade domains. Analysis indicates that coordinated policy approaches achieve 30-50% greater implementation compared to siloed initiatives with equivalent resources.
- Supply chain transformation: Moving beyond niche markets to mainstream carbon as a standard agricultural consideration. Leading food companies have committed to carbon-neutral or carbon-negative supply chains by 2030-2040, potentially creating market pull for carbon farming across hundreds of millions of hectares.
- True cost accounting: Developing valuation systems that recognize the full social and environmental costs of agricultural production. Research demonstrates that when externalities are properly valued, carbon farming systems show 20-40% higher total returns to society compared to conventional systems.
- Just transition approaches: Ensuring that carbon farming benefits are equitably distributed across farm scales and geographies. Analysis shows that targeted support for small and medium-scale producers can achieve 15-30% more total sequestration per dollar invested than programs dominated by large operations.
Conclusion
Carbon farming represents a powerful approach to address multiple interconnected challenges facing global agriculture. By enhancing the biological processes that capture carbon dioxide from the atmosphere and store it in soils and vegetation, these practices simultaneously mitigate climate change, improve soil health, enhance water management, support biodiversity, and build agricultural resilience. The evidence demonstrates that well-implemented carbon farming practices sequester significant amounts of carbon while providing additional benefits to farmers and ecosystems.
The diversity of effective approaches—from simple modifications of existing cropping systems to transformative perennial agricultures—offers implementation pathways across different agricultural contexts, scales, and geographies. While economic barriers and knowledge gaps currently limit adoption, emerging carbon markets, supportive policies, technological innovations, and growing consumer demand for climate-friendly products are creating increasingly favorable conditions for carbon farming expansion.
Looking forward, the integration of advanced practices, precision technologies, optimized genetics, and enabling policies promises to further enhance the effectiveness and adoption of carbon farming. As agriculture faces mounting pressure to reduce its environmental footprint while maintaining productivity, carbon farming offers a science-based pathway that aligns agricultural production with climate stabilization and ecosystem health—transforming agriculture from a significant contributor to climate change into a critical part of the solution.
References
- Paustian, K., Larson, E., Kent, J., Marx, E., & Swan, A. (2019). Soil C sequestration as a biological negative emission strategy. Frontiers in Climate, 1, 8.
- Fargione, J. E., Bassett, S., Boucher, T., Bridgham, S. D., Conant, R. T., Cook-Patton, S. C., Ellis, P. W., Falcucci, A., Fourqurean, J. W., Gopalakrishna, T., Gu, H., Henderson, B., Hurteau, M. D., Kroeger, K. D., Kroeger, T., Lark, T. J., Leavitt, S. M., Lomax, G., McDonald, R. I., … & Griscom, B. W. (2018). Natural climate solutions for the United States. Science Advances, 4(11), eaat1869.
- Smith, P., Soussana, J. F., Angers, D., Schipper, L., Chenu, C., Rasse, D. P., Batjes, N. H., van Egmond, F., McNeill, S., Kuhnert, M., Arias‐Navarro, C., Olesen, J. E., Chirinda, N., Fornara, D., Wollenberg, E., Álvaro‐Fuentes, J., Sanz‐Cobena, A., & Klumpp, K. (2020). How to measure, report and verify soil carbon change to realize the potential of soil carbon sequestration for atmospheric greenhouse gas removal. Global Change Biology, 26(1), 219-241.
- Bradford, M. A., Carey, C. J., Atwood, L., Bossio, D., Fenichel, E. P., Gennet, S., Fargione, J., Fisher, J. R. B., Fuller, E., Kane, D. A., Lehmann, J., Oldfield, E. E., Ordway, E. M., Rudek, J., Sanderman, J., & Wood, S. A. (2019). Soil carbon science for policy and practice. Nature Sustainability, 2(12), 1070-1072.
- Lal, R. (2018). Digging deeper: A holistic perspective of factors affecting soil organic carbon sequestration in agroecosystems. Global Change Biology, 24(8), 3285-3301.
- Powlson, D. S., Stirling, C. M., Jat, M. L., Gerard, B. G., Palm, C. A., Sanchez, P. A., & Cassman, K. G. (2014). Limited potential of no-till agriculture for climate change mitigation. Nature Climate Change, 4(8), 678-683.
- Poeplau, C., & Don, A. (2015). Carbon sequestration in agricultural soils via cultivation of cover crops – A meta-analysis. Agriculture, Ecosystems & Environment, 200, 33-41.
- Zomer, R. J., Bossio, D. A., Sommer, R., & Verchot, L. V. (2017). Global sequestration potential of increased organic carbon in cropland soils. Scientific Reports, 7(1), 15554.
- De Stefano, A., & Jacobson, M. G. (2018). Soil carbon sequestration in agroforestry systems: A meta-analysis. Agroforestry Systems, 92(2), 285-299.
- Crews, T. E., Carton, W., & Olsson, L. (2018). Is the future of agriculture perennial? Imperatives and opportunities to reinvent agriculture by shifting from annual monocultures to perennial polycultures. Global Sustainability, 1, e11.
- Machmuller, M. B., Kramer, M. G., Cyle, T. K., Hill, N., Hancock, D., & Thompson, A. (2015). Emerging land use practices rapidly increase soil organic matter. Nature Communications, 6, 6995.
- Minasny, B., Malone, B. P., McBratney, A. B., Angers, D. A., Arrouays, D., Chambers, A., Chaplot, V., Chen, Z. S., Cheng, K., Das, B. S., Field, D. J., Gimona, A., Hedley, C. B., Hong, S. Y., Mandal, B., Marchant, B. P., Martin, M., McConkey, B. G., Mulder, V. L., … & Winowiecki, L. (2017). Soil carbon 4 per mille. Geoderma, 292, 59-86.
- Searchinger, T. D., Wirsenius, S., Beringer, T., & Dumas, P. (2018). Assessing the efficiency of changes in land use for mitigating climate change. Nature, 564(7735), 249-253.
- Lehmann, J., Bossio, D. A., Kögel-Knabner, I., & Rillig, M. C. (2020). The concept and future prospects of soil health. Nature Reviews Earth & Environment, 1(10), 544-553.
- Erb, K. H., Kastner, T., Plutzar, C., Bais, A. L. S., Carvalhais, N., Fetzel, T., Gingrich, S., Haberl, H., Lauk, C., Niedertscheider, M., Pongratz, J., Thurner, M., & Luyssaert, S. (2018). Unexpectedly large impact of forest management and grazing on global vegetation biomass. Nature, 553(7686), 73-76.
If you want to learn more about Carbon Farming Systems, check out Agri AI : Smart Farming Advisor and feel free to ask any questions!
Leave a Reply