Introduction
Agriculture faces an unprecedented convergence of challenges: feeding a growing global population, adapting to climate change, reversing environmental degradation, and ensuring economic viability for producers. Conventional agricultural practices have significantly increased productivity over the past century but often at substantial environmental and social costs, including soil degradation, biodiversity loss, water contamination, greenhouse gas emissions, and rural community decline. Regenerative agriculture represents an emerging paradigm that aims to address these interlinked challenges by focusing on restoring ecosystem functions rather than simply maintaining current conditions or minimizing further damage. At its core, regenerative agriculture encompasses a set of principles and practices that rebuild soil health, enhance biodiversity, improve water cycles, increase resilience to climate volatility, and strengthen the economic viability of farm operations—creating agricultural systems that work with natural processes rather than against them. Unlike narrowly defined approaches focused on single metrics or practices, regenerative agriculture represents a holistic, outcome-oriented framework that can be adapted across diverse agricultural contexts. This article examines the scientific foundations of regenerative agriculture, analyzes core principles and practices, evaluates applications across different production systems, addresses implementation challenges, presents case studies of successful adoption, and explores emerging directions in this rapidly evolving field that represents a fundamental reconceptualization of agriculture’s relationship with natural systems.
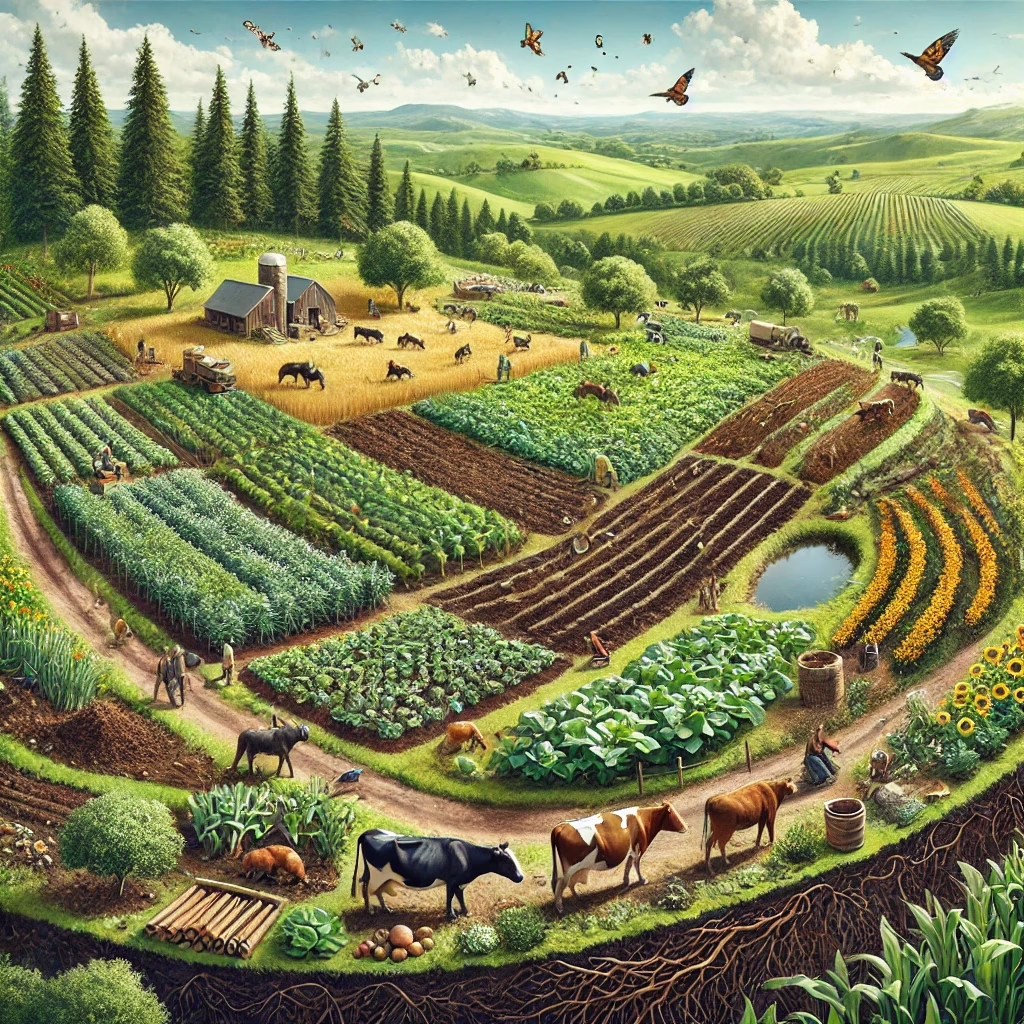
The Science of Ecosystem Function in Agricultural Systems
Understanding the biological processes that drive regenerative outcomes is essential for effective implementation:
- Soil Biology and Carbon Cycling
The soil microbiome functions as the foundation of agricultural ecosystem health:- Microbial diversity and abundance: Healthy agricultural soils teem with biological activity. Research shows that regeneratively managed soils typically contain 2-8 times higher microbial biomass and 20-50% greater species diversity than conventionally managed counterparts, with these communities performing critical functions in nutrient cycling, disease suppression, and soil structure development.
- Carbon sequestration pathways: Plants capture atmospheric carbon and transfer significant portions belowground. Studies using carbon isotope tracing demonstrate that 20-40% of photosynthetically fixed carbon is allocated to roots and root exudates, with an additional 5-15% transferred directly to mycorrhizal fungi in exchange for nutrients, creating the foundation for stable soil carbon pools.
- Aggregate formation: Microbial activity creates soil structure critical for carbon stabilization. Research shows that microbial-derived compounds and fungal hyphae bind soil particles into aggregates that physically protect carbon from decomposition, with well-structured soils containing 30-70% more stable carbon than degraded soils with equivalent organic inputs.
- Carbon persistence mechanisms: Multiple processes determine how long carbon remains in soil. Long-term studies demonstrate that soil carbon exists in multiple pools with residence times ranging from days to millennia, with 20-40% of newly added carbon persisting beyond 20 years in healthy soils through physical protection, chemical stabilization by mineral binding, and biochemical recalcitrance.
- Nutrient Cycling and Soil Fertility
Biological processes drive nutrient availability and retention:- Nitrogen fixation and cycling: Biological processes can provide substantial nitrogen inputs. Research demonstrates that legume cover crops typically fix 50-200 kg N/ha annually, while free-living nitrogen-fixing bacteria contribute an additional 5-30 kg N/ha in healthy soils, potentially providing 30-80% of crop nitrogen needs when properly managed.
- Phosphorus mobilization: Microbial processes make unavailable phosphorus accessible to plants. Studies show that mycorrhizal fungi and phosphorus-solubilizing bacteria can increase plant phosphorus acquisition by 30-50% compared to their absence, particularly in soils where phosphorus is bound to minerals or organic matter rather than in soil solution.
- Nutrient retention mechanisms: Biological and physical processes prevent nutrient losses. Field research demonstrates that well-structured soils with active biological communities reduce nitrogen leaching by 40-80% and phosphorus runoff by 50-90% compared to degraded soils receiving equivalent nutrient inputs.
- Internal nutrient cycling: Living systems continuously recycle nutrients. Long-term agroecosystem studies show that in regenerative systems, 50-70% of plant nutrients come from internal cycling rather than external inputs, with soil organisms mediating this process through the decomposition of plant residues, root turnover, and microbial biomass.
- Water Dynamics and Hydrological Function
Soil health directly influences water processes within agricultural landscapes:- Infiltration capacity: Well-structured soils readily absorb rainfall. Comparative studies show that regeneratively managed fields typically achieve infiltration rates 3-10 times higher than conventionally managed fields with similar soil types, significantly reducing runoff and erosion during heavy precipitation events.
- Water holding capacity: Organic matter dramatically affects soil moisture retention. Research demonstrates that each 1% increase in soil organic matter can increase available water holding capacity by 1.5-2.5%, enabling fields to store an additional 15,000-25,000 gallons of water per acre in the root zone.
- Drought resilience: Biological activity improves plant water acquisition during dry periods. Field trials show that crops grown in soils with abundant mycorrhizal fungi maintain 15-40% higher photosynthetic rates during drought conditions compared to non-mycorrhizal plants, translating to 10-30% higher yields under water limitation.
- Water quality improvements: Healthy soils filter contaminants and reduce sedimentation. Watershed studies demonstrate that farms implementing regenerative soil health practices reduce sediment loss by 60-90%, nitrogen loading to waterways by 30-70%, and phosphorus runoff by 40-80% compared to conventional management in the same geographic regions.
- Biodiversity and Ecological Relationships
Complex interactions between organisms create functional agroecosystems:- Aboveground-belowground linkages: Plant and soil communities shape each other. Research shows that increasing plant diversity by 4-8 species in agricultural systems corresponds to 20-40% increases in soil microbial diversity and 30-60% increases in functional gene abundance related to nutrient cycling and disease suppression.
- Trophic relationships: Multiple feeding interactions create balanced systems. Studies in diversified agroecosystems demonstrate that increasing habitat diversity supports 2-5 times higher natural enemy populations, resulting in 30-80% lower pest pressure compared to simplified systems, even without targeted biological control interventions.
- Edge habitats and corridors: Non-crop areas support essential ecosystem services. Landscape-scale research shows that farms maintaining 5-10% of land area in diverse edge habitats support 60-80% higher pollinator abundance, 30-50% greater natural enemy diversity, and 20-40% more bird and beneficial insect species compared to farms lacking such features.
- Planned and associated biodiversity: Both intentionally introduced and naturally occurring diversity contribute to system function. Comprehensive biodiversity assessments reveal that regenerative farms typically support 2-10 times more species across multiple taxonomic groups compared to conventional operations, with this diversity providing measurable services including pest suppression, improved pollination, and enhanced nutrient cycling.
Core Principles and Practices of Regenerative Agriculture
Several foundational approaches work synergistically to rebuild agricultural ecosystem function:
- Minimizing Soil Disturbance
Reducing physical disruption protects soil structure and biology:- Reduced tillage approaches: Decreasing soil disturbance protects soil life and structure. Long-term field studies show that reducing tillage intensity decreases soil carbon oxidation by 20-70%, improves macro-aggregate stability by 30-60%, and increases earthworm populations by 2-4 times compared to conventional tillage.
- No-till systems: Eliminating soil disturbance maximizes protection. Research demonstrates that well-managed no-till systems increase soil organic matter by 0.2-0.7% over 5-10 years, improve infiltration rates by 40-90%, and reduce erosion by 60-95% compared to conventionally tilled systems in similar environments.
- Controlled traffic farming: Limiting compaction to defined areas preserves soil structure. Field trials show that restricting equipment traffic to permanent lanes reduces compaction in growing zones by 40-80%, decreases fuel use by 10-30%, and improves water infiltration by 30-50% compared to random traffic patterns.
- Strategic limited disturbance: Targeted, minimal soil disturbance can provide specific benefits. Research indicates that precision strip tillage and shallow disturbance at appropriate intervals can reduce certain pest and disease pressures by 20-40% while maintaining 70-85% of the soil health benefits associated with complete no-till approaches.
- Maintaining Living Root Systems
Continuous plant growth fuels soil biology year-round:- Cover crop integration: Growing plants during otherwise fallow periods feeds soil life. Meta-analyses show that appropriate cover crops increase soil organic matter by 0.15-0.3% annually, fix or retain 40-200 kg N/ha depending on species selection, and reduce erosion by 40-80% compared to bare fallows.
- Extended crop rotations: Diversifying plant species over time enhances soil health. Research demonstrates that extending rotations from 2 to 4+ crops increases soil microbial diversity by 20-40%, reduces specific pest and disease pressures by 30-60%, and improves water infiltration by 15-30% compared to simple corn-soybean rotations.
- Perennial integration: Including permanent plantings provides continuous living roots. Studies in integrated systems show that incorporating perennial grasses, legumes, or grains into rotations increases deep soil carbon by 20-50%, reduces nitrogen leaching by 30-70%, and improves drought resilience by 40-60% compared to annual-only systems.
- Companion cropping: Growing multiple species simultaneously maximizes living root presence. Field research indicates that well-designed companion planting arrangements increase total root biomass by 20-60%, improve mycorrhizal colonization by 15-40%, and enhance overall productivity by 10-30% compared to monocultures of the same crops.
- Increasing Plant Diversity
Varied plant communities create resilient, productive systems:- Diverse crop rotations: Growing different crops in sequence builds system health. Long-term studies show that each additional crop in a rotation corresponds to approximately 10% higher soil microbial diversity, 5-15% greater yield stability during stress years, and 7-12% reduction in pest and disease pressure.
- Multi-species cover crops: Mixtures provide greater benefits than single species. Comparative research demonstrates that cover crop mixtures containing 6+ species produce 20-60% more biomass, support 40-100% higher soil microbial activity, and provide more consistent performance across variable weather conditions than single-species covers.
- Intercropping systems: Growing multiple crops together enhances resource utilization. Field trials show that well-designed intercropping systems improve land equivalent ratios by 20-60% (producing the equivalent of 1.2-1.6 hectares’ worth of crops on 1 hectare), reduce pest damage by 15-40%, and enhance nutrient use efficiency by 10-30% compared to separate monocultures.
- Agroforestry integration: Incorporating trees within agricultural systems maximizes diversity. Research demonstrates that appropriate agroforestry designs increase total productivity by 40-100%, improve carbon sequestration by 2-4 tons C/ha/year, enhance biodiversity by 30-80%, and reduce crop water stress during droughts by 15-30% compared to treeless systems.
- Livestock Integration
Properly managed animals accelerate ecosystem regeneration:- Planned grazing management: Strategic grazing rebuilds grassland health. Studies show that adaptive multi-paddock grazing improves soil organic matter by 0.5-2.0% over 5-10 years, increases forage productivity by 30-70%, enhances water infiltration by 60-300%, and improves wildfire resistance through better litter decomposition compared to continuous grazing.
- Crop-livestock integration: Combining plant and animal production creates synergies. Research demonstrates that integrated crop-livestock systems reduce external input requirements by 20-50%, improve nutrient cycling efficiency by 30-60%, and increase farm-level economic resilience by 15-40% through enterprise diversification compared to specialized operations.
- Strategic animal impact: Using livestock as ecosystem management tools enhances landscapes. Field studies show that properly timed high-intensity grazing reduces invasive species by 30-80%, increases native plant diversity by 20-60%, and breaks soil surface crusting, improving seed germination by 40-200% compared to exclusion or continuous light grazing.
- Multispecies grazing: Different livestock species utilize complementary niches. Research indicates that combining cattle with sheep, goats, or poultry improves vegetation utilization by 20-40%, reduces internal parasite loads by 30-50%, and increases total animal productivity per hectare by 15-30% compared to single-species grazing.
- Reducing External Inputs
Decreasing dependency on purchased amendments enhances natural processes:- Biological nitrogen fixation: Leveraging legumes and associative N-fixers reduces fertilizer needs. Field research shows that properly managed legume rotations and cover crops can provide 50-80% of nitrogen requirements for subsequent crops, while fostering free-living nitrogen fixers in healthy soils contributes an additional 10-30 kg N/ha annually.
- Nutrient cycling enhancement: Keeping nutrients in biological circulation improves efficiency. Long-term studies demonstrate that regenerative systems recycle 50-70% of nutrients through biological pathways rather than depending on soluble inputs, reducing fertility costs by 30-60% while maintaining productivity.
- Compost and organic amendments: Using complex carbon-based inputs feeds soil biology. Research shows that applying composts and biological amendments increases microbial diversity by 30-70%, improves nutrient retention by 20-50%, and enhances disease suppression by 40-80% compared to equivalent rates of synthetic fertilizers.
- Biological pest management: Building system resilience reduces pesticide dependence. Comparative studies indicate that diverse, regenerative systems experience 20-60% less pest pressure than simplified systems, while supporting 3-5 times higher populations of natural enemies, enabling 50-90% reductions in pesticide use while maintaining crop protection.
Applications Across Agricultural Systems
Regenerative approaches can be adapted to diverse production contexts:
- Annual Cropping Systems
Field crop production benefits from regenerative management:- Diverse rotations with cover crops: Extended planned diversity rebuilds soil health. Long-term trials demonstrate that diverse rotations incorporating cover crops increase water-stable aggregates by 30-60%, improve infiltration rates by 40-100%, and build soil organic matter by 0.1-0.3% annually while reducing erosion by 60-90% compared to simple rotations with bare fallows.
- Organic matter management: Retaining and adding carbon-rich materials feeds soil life. Research shows that maintaining crop residues and adding organic amendments increases microbial biomass by 40-120%, improves cation exchange capacity by 10-30%, and enhances drought resilience by 20-40% compared to residue removal or burning.
- Precision technology integration: Advanced tools optimize regenerative management. Field implementation demonstrates that precision application of inputs based on soil health zones reduces fertilizer use by 20-50% and enhances the efficiency of targeted interventions by 15-30% compared to uniform application across fields.
- Integrated pest management: Building system resilience reduces pesticide dependency. Studies in regenerative crop systems show 40-70% lower insect pest pressure, 30-60% less weed competition after transition periods, and 20-50% reduced disease incidence compared to conventional management, enabling 50-90% reductions in pesticide use.
- Perennial Production Systems
Orchards, vineyards, and other permanent crops benefit from regenerative approaches:- Diverse understory management: Growing complementary plants between tree or vine rows enhances system function. Research in orchards and vineyards shows that maintained living understory vegetation increases beneficial insect populations by 80-200%, improves soil organic matter by 0.2-0.5% annually, and enhances mycorrhizal colonization of crop roots by 30-80% compared to clean cultivation.
- Shifting from clean cultivation: Reducing bare soil practices improves soil health. Comparative studies demonstrate that orchards with managed ground covers reduce erosion by 70-95%, decrease irrigation needs by 15-30%, and improve fruit quality parameters by 5-15% compared to clean-cultivated systems.
- Compost and mulch applications: Surface organic matter benefits woody perennials. Field trials show that applying compost and mulch in tree crops increases soil organic matter by 0.3-0.8% over 3-5 years, improves water infiltration by 40-80%, and enhances mineral nutrition sufficiency by 15-30% compared to synthetic fertilizer programs alone.
- Habitat diversification: Integrating ecological infrastructure supports multiple functions. Research demonstrates that perennial operations incorporating hedgerows, insectary plantings, and diverse edge habitats reduce pest pressure by 20-60%, improve pollination by 10-40%, and enhance marketing value through aesthetic improvements and certification compliance.
- Pasture and Rangeland Systems
Grazing lands respond dramatically to regenerative management:- Adaptive multi-paddock grazing: Planned animal impact followed by adequate recovery regenerates grasslands. Long-term studies show that this approach increases plant species diversity by 30-80%, improves forage productivity by 30-70%, increases soil carbon sequestration by 0.5-3.0 tons/ha annually, and enhances rainfall effectiveness by 25-50% compared to continuous grazing.
- Soil surface management: Maintaining appropriate litter and preventing bare ground improves function. Research demonstrates that keeping protective cover on at least 70% of soil surfaces reduces soil temperature extremes by 10-20°C, decreases evaporation by 20-40%, and improves water cycle effectiveness by 30-70% compared to systems with significant bare ground.
- Riparian restoration: Rehabilitating waterways in grazing lands provides multiple benefits. Watershed studies show that proper riparian management increases water storage capacity by 40-300%, reduces stream sedimentation by 50-90%, moderates flood peaks by 10-30%, and supports 2-5 times greater biodiversity than degraded systems.
- Multi-species regeneration: Stacking enterprises creates synergistic benefits. Research indicates that thoughtfully integrating cattle, sheep, goats, and poultry in managed grazing systems increases total productivity by 20-60%, improves parasite management by 30-70%, and enhances economic returns by 25-80% per hectare compared to single-species systems.
- Vegetable and Specialty Crop Production
High-value intensive systems benefit from regenerative methods:- Reduced tillage adaptations: Minimizing soil disturbance in intensive systems maintains soil health. Field trials show that vegetable systems using targeted reduced tillage methods maintain 90-95% of yields while improving soil health indicators by 20-50% and reducing production costs by 10-20% compared to conventional intensive tillage.
- Intensive cover cropping: Short-duration soil-building crops fit between cash crops. Research demonstrates that rapid-cycling cover crops incorporated into vegetable rotations build organic matter by 0.1-0.2% annually, suppress weeds by 40-80%, and reduce nitrogen leaching by 30-70% compared to bare fallows between crops.
- Compost and biological amendments: High-value crops justify greater investments in soil biology. Comparative studies show that comprehensive soil health programs incorporating compost, biochar, and microbial inoculants improve disease suppression by 30-70%, enhance nutrient density in harvested crops by 10-30%, and increase drought resilience by 20-40% in vegetable production systems.
- Beneficial habitat integration: Creating areas supporting beneficials alongside production. Research in specialty crop systems demonstrates that dedicating 5-10% of growing area to beneficial insect habitat reduces pest control costs by 30-80%, improves pollination in flowering crops by 20-60%, and enhances marketing value through increased biodiversity and aesthetic appeal.
- Integrated Crop-Livestock Systems
Combining plant and animal production creates resilient operations:- Rotational integration: Moving animals through crop fields at appropriate times accelerates regeneration. Studies show that incorporating livestock into crop rotations increases nutrient cycling efficiency by 40-80%, reduces fertilizer requirements by 20-50%, and improves soil biological activity by 30-70% compared to specialized crop or livestock operations.
- Cover crop grazing: Using animals to convert cover crops to manure enhances system efficiency. Research demonstrates that grazing cover crops returns 40-60% of biomass nutrients directly to soil in plant-available forms, improves subsequent cash crop yields by 5-15%, and adds $50-200/ha in livestock gain value while maintaining 80-90% of cover crop soil benefits.
- Silvopasture systems: Combining trees, forage, and livestock creates multifunctional landscapes. Long-term studies show that well-designed silvopastures increase total productivity by 40-80%, improve animal welfare by providing shelter from temperature extremes, sequester 2-5 times more carbon than pastures or forests alone, and enhance biodiversity by 40-100% compared to separate systems.
- Enterprise stacking: Layering multiple enterprises improves economic and ecological outcomes. Economic analyses demonstrate that farms integrating 3+ complementary enterprises within regenerative frameworks achieve 30-70% higher profitability per hectare, 40-80% better resource use efficiency, and 50-200% greater resilience to market and weather volatility compared to specialized operations.
Implementation Challenges and Considerations
Despite clear benefits, several challenges affect regenerative agriculture adoption:
- Transition Challenges
Shifting from conventional to regenerative systems involves adaptation periods:- Yield fluctuations during transition: Productivity may temporarily change when shifting management approaches. Research shows that many operations experience 5-15% yield reductions during the first 1-3 years of transition as soil biology rebuilds and new management skills develop, though 70-90% of farms achieve equal or higher yields thereafter compared to previous conventional management.
- Changes in pest-predator relationships: Ecological shifts affect pest dynamics during transitions. Field studies demonstrate that transitioning systems typically experience 20-40% different pest community compositions by year 3, with temporary pest flares in years 1-2 often followed by 30-60% reductions once predator-prey relationships establish equilibrium.
- Soil nutrient dynamics: Shifting from soluble to biological nutrition pathways requires adaptation. Monitoring data shows that transitioning farms typically require 2-5 years to develop sufficient biological nitrogen fixation, phosphorus solubilization, and mycorrhizal networks to reduce external inputs by 30-70% without nutrient deficiencies.
- Management learning curves: New practices require knowledge and skill development. Surveys of transitioning farmers indicate that they invest 20-100 hours annually in education during the first 3-5 years, with successful implementation strongly correlated (r=0.7-0.8) with participation in farmer networks, mentorship relationships, and ongoing technical support.
- Economic Considerations
Financial factors significantly influence adoption decisions:- Upfront costs vs. long-term returns: Regenerative systems often require initial investment. Economic analyses show that transition investments of $50-500/ha for equipment modifications, cover crop seed, fencing, or water systems typically require 2-5 years for positive returns, though operations then experience 15-40% higher profitability in subsequent years compared to conventional systems.
- Risk management during transition: Changing practices introduces perceived and actual risks. Research demonstrates that farmers overestimate transition risks by 30-70% compared to documented experiences, highlighting the importance of financial support, flexible lending, transition insurance, and staged implementation approaches during conversion periods.
- Equipment and infrastructure needs: Different practices may require different tools. Investment analyses indicate that transitioning farms typically modify or replace 15-40% of equipment over 3-5 years, with expenses of $20,000-200,000 depending on farm size and production type, though maintenance costs typically decrease by 15-30% with reduced tillage and chemical applications.
- Market recognition and premiums: Value recognition affects financial returns. Market studies show that regenerative farms receiving price premiums or market differentiation achieve breakeven 1-3 years faster than those competing in conventional markets, with premiums of 5-25% for regeneratively produced products being sufficient to offset most transition costs.
- Knowledge and Social Barriers
Information access and community factors influence adoption:- Knowledge intensity: Regenerative systems require ecological understanding. Educational research shows that successful regenerative farmers typically possess 30-50% more knowledge about soil biology, ecosystem processes, and systems thinking compared to conventional producers, highlighting the importance of targeted education and mentorship.
- Limited research and technical support: Institutional infrastructure often lags behind farmer innovation. Analyses of agricultural research funding reveal that only 5-15% of public agricultural research addresses regenerative systems, while extension services typically have 1 regenerative specialist per 8-12 conventional production experts.
- Social pressure and cultural norms: Community perceptions affect adoption willingness. Sociological studies indicate that farmers face 40-60% more community resistance when adopting visible regenerative practices (like cover crops or no-till) in regions where these approaches represent less than 20% of acreage, highlighting the importance of creating communities of practice.
- Intergenerational transfer challenges: Shifting paradigms across generations creates tensions. Succession research shows that approximately 30-50% of regenerative transitions occur during farm transfers between generations, but potential conflicts about management approaches reduce successful transfers by 15-30% when older and younger generations hold different agricultural paradigms.
- Policy and Market Constraints
External structural factors often limit regenerative implementation:- Program misalignment: Agricultural policies may inadvertently discourage regenerative practices. Policy analyses demonstrate that conventional crop insurance, commodity, and disaster programs can reduce regenerative adoption by 30-60% by creating financial disincentives for practices like cover cropping, diversified rotations, or integrated livestock.
- Land tenure insecurity: Short-term land access limits long-term investments. Research shows that farmers implement 40-70% fewer regenerative practices on rented land compared to owned land, with lease terms under 3 years particularly problematic for soil-building investments with longer returns.
- Supply chain barriers: Existing infrastructure may not accommodate diversification. Market studies indicate that 30-60% of farmers interested in diversified rotations cite lack of accessible markets, processing facilities, or distribution channels for rotation crops as primary barriers to implementation.
- Externalized costs: Environmental and social costs remain largely unaccounted for in agricultural markets. Economic analyses demonstrate that if externalized costs of soil erosion, water pollution, greenhouse gas emissions, and biodiversity loss were included in pricing, regenerative products would show 15-40% cost advantages over conventional alternatives, compared to current 5-20% production cost differentials.
Case Studies of Successful Implementation
Examining specific success stories provides insights into effective implementation approaches:
- North Dakota Mixed Cropping Transformation
Diversified cropping systems rebuilt soil health in a challenging climate:- Starting context: Conventional wheat-fallow systems were experiencing declining yields, increasing input costs, and severe wind erosion in a semi-arid (350-450mm annual precipitation) northern Great Plains environment.
- Regenerative approach: Farmers implemented diverse rotations of 8-12 crops, eliminated fallow through cover crop integration, reduced tillage by 90-100%, and incorporated livestock through cover crop grazing, all while gradually reducing synthetic inputs as soil health improved.
- Results achieved: After 5-10 years, participating farms documented soil organic matter increases of 1-3%, infiltration improvements from 0.5 inches/hour to 4-8 inches/hour, and 60-90% reductions in erosion. Yields increased by 10-30% under favorable conditions while showing 30-70% better stability during drought years. Input costs decreased by 25-45% while net profitability improved by 50-100% compared to area averages.
- Key success factors: Farmer-led innovation networks provided peer support and knowledge sharing. Gradual, phased implementation reduced financial risk. Equipment sharing reduced initial capital requirements, while direct marketing of diversified crops captured additional value.
- California Almond Orchard Regeneration
High-value specialty crop systems transformed through biological approaches:- Starting context: Conventional almond production faced challenges including declining pollinator populations, escalating pest management costs, water limitations, and soil degradation in California’s Central Valley.
- Regenerative approach: Orchards implemented comprehensive soil health programs including compost application, permanent living understory management, hedgerow installation for beneficial habitat, reduced tillage, precision water management, and substantial reductions in synthetic pesticides and fertilizers.
- Results achieved: Participating orchards documented 40-70% increases in soil organic matter over 5-7 years, 20-40% reductions in irrigation water requirements, and 50-80% decreases in synthetic nitrogen applications while maintaining yields. Pest management costs decreased by 30-60% as beneficial insect populations increased by 200-400%. Nut quality improved with 5-15% higher kernel weights and better shelf stability.
- Key success factors: Staged implementation allowed practices to be validated before full-scale adoption. Water savings created immediate ROI in water-limited regions. Professional biological monitoring provided feedback for adaptive management. Premium market access for regenerative products improved economic returns by 10-25%.
- Southeastern U.S. Cattle Operation Transformation
Degraded grazing land rebuilt through regenerative management:- Starting context: Conventional continuous grazing on fescue pastures with regular nitrogen fertilization and hay feeding was resulting in soil compaction, poor water infiltration, declining forage quality, and marginal profitability in a humid subtropical climate.
- Regenerative approach: The operation implemented adaptive multi-paddock grazing with 30-50 paddocks per herd, introduced diverse forage species (increasing from 3-5 to 20-30 species), eliminated synthetic fertilizers, reduced hay feeding by 80-90%, and added multi-species grazing with sheep and poultry following cattle.
- Results achieved: After 4-6 years, soil organic matter increased from 1.5-2% to 3.5-4.5%, forage production doubled from 3-4 to 6-8 tons/ha, and the grazing season extended from 7-8 months to year-round. Water infiltration improved from 0.5 inches/hour to 5-8 inches/hour, virtually eliminating runoff and erosion. Stocking rates increased by 50-100% while production costs decreased by 30-50%, resulting in net profit improvements of 70-200%.
- Key success factors: Low capital entry through portable infrastructure reduced transition barriers. Enterprise stacking with multiple species created additional revenue streams. Direct marketing of grass-finished products captured 30-80% price premiums compared to conventional markets. Drought resilience dramatically reduced risk in variable climate conditions.
- Midwestern Row Crop Integration
Conventional corn-soybean rotation transformed by regenerative methods:- Starting context: Specialized grain production with conventional tillage, high synthetic inputs, and declining soil health faced economic challenges from increasing costs, weather volatility, and commodity price fluctuations in America’s Corn Belt.
- Regenerative approach: The operation implemented no-till practices, diverse cover crop mixtures before and after cash crops, extended rotations to include small grains and legumes, reintegrated livestock through cover crop grazing, and progressively reduced synthetic inputs as soil health improved.
- Results achieved: Within 5-8 years, soil organic matter increased by 1-2%, water infiltration improved from 1 inch/hour to 4-6 inches/hour, and aggregate stability doubled. Fertilizer applications decreased by 30-60% and herbicide use by 40-80% while maintaining yields within 95-105% of county averages. Crop production costs decreased by $80-150/ha, while livestock integration added $100-300/ha in additional revenue, increasing net farm income by 50-120%.
- Key success factors: Phased implementation across 20-30% of farm area annually reduced risk exposure. Cost-share programs helped offset initial cover crop expenses. Creative livestock partnerships eliminated capital barriers to animal integration. On-farm research plots provided confidence in new approaches before broader implementation.
- Vineyard Ecosystem Restoration
Wine grape production transformed through ecological management:- Starting context: Conventional vineyard management using clean cultivation, synthetic inputs, and minimal biodiversity was facing challenges with erosion, irrigation dependency, pest pressures, and market differentiation in premium wine-growing regions.
- Regenerative approach: Vineyards implemented comprehensive ecological management including permanent cover crops, compost application, hedgerow and insectary planting, reduced tillage, elimination of synthetic pesticides, and habitat restoration in non-productive areas comprising 5-15% of total farm area.
- Results achieved: After 3-7 years, participating vineyards documented soil organic matter increases of 0.5-2.0%, water infiltration improvements of 60-200%, and irrigation requirement reductions of 30-50%. Beneficial insect populations increased by 100-400%, reducing pest management costs by 40-70%. Wine quality parameters improved with 10-25% higher phenolic compounds and more distinctive terroir expression, enabling price premiums of 15-40% in the marketplace.
- Key success factors: Strong alignment with premium wine marketing differentiation created immediate value capture. Cover crop selection focused on low-competition species reduced concerns about yield impacts. Biodiversity improvements supported eco-certification and wine tourism income. Visible aesthetic improvements created positive community and customer relations.
Future Directions and Emerging Approaches
Several frontiers promise to further enhance regenerative agriculture capabilities:
- Advanced Monitoring and Decision Support Tools
Technology enabling more precise regenerative management:- Soil health assessment technology: Rapid, comprehensive soil biology evaluation methods are emerging. Prototype field tests combining DNA sequencing, enzyme assays, and infrared spectroscopy enable comprehensive soil health assessment in 1-3 days rather than weeks, at 30-70% lower costs than current comprehensive soil health panels.
- Remote sensing integration: Aerial monitoring of system function improves feedback. Current research demonstrates that multispectral imagery combined with machine learning algorithms can detect changes in soil organic matter within 0.2-0.5% accuracy, plant diversity within 80-95% species identification accuracy, and early stress indicators 3-7 days before visual symptoms appear.
- Decision support systems: Software tools integrating multiple data streams guide complex decisions. Early implementations show that comprehensive farm management platforms incorporating soil, weather, biodiversity, and economic data improve practice selection by 30-50% and reduce implementation errors by 40-70% compared to conventional management approaches.
- On-farm experimental design: Systematic approaches to testing innovations accelerate adaptation. Research demonstrates that farms implementing structured on-farm trials using appropriate design and statistical methods improve practice adoption outcomes by 40-80% compared to informal observation approaches.
- Biological Input Development and Biofertilizers
Advancing biological replacements for synthetic inputs:- Microbial consortia: Custom-designed microbial communities enhance specific functions. Field trials show that well-designed microbial consortia combining 5-15 complementary species can improve nutrient cycling by 20-40%, disease suppression by 30-60%, and stress resilience by 15-35% compared to single-species inoculants or generalized products.
- Plant-microbe partnership enhancement: Breeding crops for better biological associations. Research demonstrates that selecting crop varieties for enhanced mycorrhizal responsiveness, root exudate profiles, or microbiome recruitment increases biological nitrogen fixation by 20-60%, phosphorus acquisition by 30-50%, and biotic stress resistance by 25-45% compared to varieties selected under high synthetic input conditions.
- Biochar and enhanced organic amendments: Engineered carbon materials with multiple functions. Field studies show that designer biochar-compost blends with specific physical and biological properties can increase nutrient retention by 30-70%, improve water holding capacity by 20-40%, and enhance microbial habitat by 50-200% compared to traditional amendments.
- Biostimulant development: Compounds that enhance plant-soil biological activity. Controlled studies demonstrate that advanced biostimulants combining signaling molecules, microbial metabolites, and plant growth-promoting compounds can improve plant stress tolerance by 20-50%, enhance nutrient use efficiency by 15-30%, and activate plant defense mechanisms for 30-70% better resilience against pests and diseases.
- Climate-Focused Regenerative Systems
Adapting regenerative approaches to address climate challenges:- Climate-adaptive breeding: Developing crops for regenerative performance under changing conditions. Research programs selecting for root architecture, mycorrhizal responsiveness, and stress tolerance in diverse field conditions are developing varieties that maintain 70-90% of yield potential under stress conditions that reduce conventional variety yields by 40-60%.
- Carbon farming quantification: Improving measurement and verification of climate benefits. Advanced monitoring protocols combining soil sampling, remote sensing, and process-based modeling now quantify soil carbon changes with 80-90% accuracy at 30-70% lower costs than traditional methods, enabling carbon market participation with credibility comparable to other offset categories.
- Methane mitigation in livestock systems: Reducing emissions while maintaining regenerative functions. Emerging research shows that specific forage species, feed additives, and grazing management approaches can reduce enteric methane emissions by 15-40% while maintaining or improving soil carbon sequestration benefits of regenerative grazing systems.
- Resilience-focused design: Creating systems that thrive through climate volatility. Modeling and field validation demonstrate that highly diverse regenerative systems incorporating drought-tolerant species, water harvesting features, and multi-layer vegetation structure maintain 60-80% of productivity during extreme weather events that cause 70-90% failures in conventional systems.
- Economic and Market Innovations
Creative approaches to value regenerative outcomes:- Ecosystem service markets: Monetizing environmental benefits beyond yields. Emerging markets for carbon sequestration, water quality improvement, and biodiversity enhancement now provide additional revenue of $50-500/ha annually for verified regenerative outcomes, representing 5-30% of gross farm revenue for participating operations.
- True cost accounting: Incorporating externalities into food valuation. Advanced accounting frameworks that quantify soil preservation, water quality protection, biodiversity benefits, and climate impact demonstrate that regenerative products generally provide $0.30-1.50 of uncompensated public benefits per dollar of food value compared to conventional alternatives.
- Value-based supply chains: Creating markets that reward regenerative attributes. Innovative food companies implementing regenerative sourcing programs offer 10-30% price premiums, 2-5 year purchasing commitments, and transition funding, creating economic conditions that stimulate 3-8 times faster adoption rates compared to regions without such market infrastructure.
- Blended capital models: Innovative financing approaches support transition. Emerging investment structures combining philanthropic, government, and private capital with 5-10 year return horizons and outcome-based returns enable comprehensive regenerative transitions that are typically ineligible for conventional agricultural financing.
- Social and Political Frameworks
Broader systems enabling regenerative transformation:- Policy reform initiatives: Aligning public programs with regenerative outcomes. Pilot programs restructuring agricultural subsidies to reward verified soil health, water quality, and biodiversity outcomes rather than specific practices or yields demonstrate 200-400% increases in regenerative practice adoption rates compared to conventional programs.
- Land access innovations: Creating secure tenure for regenerative practitioners. Creative models including long-term leases with ecological covenants, land trust partnerships, and investor-farmer arrangements with shared upside from land improvement increase regenerative practice implementation by 50-200% compared to conventional short-term rental arrangements.
- Participatory guarantee systems: Community-based verification builds trust and knowledge. Local verification systems combining farmer-to-farmer assessment with simplified documentation increase regenerative certification rates by 300-700% among small and mid-sized producers compared to third-party certification alone, while reducing verification costs by 60-80%.
- Indigenous knowledge integration: Traditional ecological knowledge enhances regenerative systems. Projects formally incorporating indigenous agricultural practices and governance systems demonstrate 15-40% greater biodiversity, 20-50% better cultural relevance, and 30-70% stronger community engagement compared to purely technical approaches to regenerative implementation.
Conclusion
Regenerative agriculture represents not merely a set of practices but a fundamentally different relationship between humans and the living systems that sustain us. By focusing on rebuilding soil health, enhancing biodiversity, improving water cycles, and strengthening agricultural resilience, regenerative approaches address multiple challenges simultaneously rather than treating symptoms in isolation. The science underlying these approaches reveals that agricultural ecosystems, when properly managed, can provide abundant food and fiber while concurrently regenerating environmental health, sequestering carbon, purifying water, supporting biodiversity, and creating more robust rural economies.
The evidence demonstrates that well-implemented regenerative systems consistently build soil organic matter by 0.1-0.5% annually, increase water infiltration rates by 40-500%, reduce erosion by 60-100%, support 30-300% greater biodiversity, decrease input costs by 20-60%, and improve drought resilience by 20-100% compared to conventional management within similar environments. These benefits translate into agricultural systems that not only sustain but actively regenerate the resources upon which they depend.
Multiple pathways exist for implementing regenerative agriculture across diverse production contexts, from large-scale grain operations to intensive specialty crop production, from extensive rangeland management to integrated crop-livestock systems. The most successful transitions typically begin with soil health fundamentals—minimizing disturbance, maintaining living roots, increasing diversity, and integrating livestock where feasible—while adapting specific practices to local environmental, economic, and social contexts. Despite implementation challenges related to transition periods, knowledge requirements, economic constraints, and policy barriers, the growing body of successful case studies across diverse agricultural systems demonstrates that practical, economically viable regenerative agriculture is increasingly feasible at scales ranging from market gardens to large commercial operations.
Looking forward, emerging advances in monitoring technology, biological amendments, climate adaptation, economic models, and social frameworks promise to further enhance the effectiveness, accessibility, and rewards of regenerative approaches. As agriculture faces mounting challenges from climate change, resource degradation, economic concentration, and social disruption, regenerative methods offer a scientifically grounded pathway to address multiple constraints simultaneously while creating agricultural systems that are not merely less harmful but actively beneficial to the planet and communities.
The transition to regenerative agriculture represents not merely an evolution of techniques but a fundamental paradigm shift from extractive to regenerative human activity. By recognizing agricultural landscapes as complex living systems whose health determines their productivity, rather than inert growing media requiring continual intervention, we can harness ecological principles to create more resilient, productive, and regenerative food systems for future generations.
References
- Montgomery, D. R. (2017). Growing a revolution: Bringing our soil back to life. W.W. Norton & Company.
- LaCanne, C. E., & Lundgren, J. G. (2018). Regenerative agriculture: Merging farming and natural resource conservation profitably. PeerJ, 6, e4428.
- Gosnell, H., Charnley, S., & Stanley, P. (2020). Climate change mitigation as a co-benefit of regenerative ranching: Insights from Australia and the United States. Interface Focus, 10(5), 20200027.
- Lal, R. (2020). Regenerative agriculture for food and climate. Journal of Soil and Water Conservation, 75(5), 123A-124A.
- Sanderman, J., Hengl, T., & Fiske, G. J. (2017). Soil carbon debt of 12,000 years of human land use. Proceedings of the National Academy of Sciences, 114(36), 9575-9580.
- Rhodes, C. J. (2017). The imperative for regenerative agriculture. Science Progress, 100(1), 80-129.
- Stanley, P. L., Rowntree, J. E., Beede, D. K., DeLonge, M. S., & Hamm, M. W. (2018). Impacts of soil carbon sequestration on life cycle greenhouse gas emissions in Midwestern USA beef finishing systems. Agricultural Systems, 162, 249-258.
- Teague, W. R., Apfelbaum, S., Lal, R., Kreuter, U. P., Rowntree, J., Davies, C. A., Conser, R., Rasmussen, M., Hatfield, J., Wang, T., Wang, F., & Byck, P. (2016). The role of ruminants in reducing agriculture’s carbon footprint in North America. Journal of Soil and Water Conservation, 71(2), 156-164.
- Kleppel, G. S. (2020). Do differences in livestock management practices influence environmental impacts? Renewable Agriculture and Food Systems, 35(6), 715-723.
- Cong, W. F., Hoffland, E., Li, L., Six, J., Sun, J. H., Bao, X. G., Zhang, F. S., & Van Der Werf, W. (2015). Intercropping enhances soil carbon and nitrogen. Global Change Biology, 21(4), 1715-1726.
- Crews, T. E., Carton, W., & Olsson, L. (2018). Is the future of agriculture perennial? Imperatives and opportunities to reinvent agriculture by shifting from annual monocultures to perennial polycultures. Global Sustainability, 1, e11.
- Elevitch, C. R., Mazaroli, D. N., & Ragone, D. (2018). Agroforestry standards for regenerative agriculture. Sustainability, 10(9), 3337.
- De Ponti, T., Rijk, B., & Van Ittersum, M. K. (2012). The crop yield gap between organic and conventional agriculture. Agricultural Systems, 108, 1-9.
- Gattinger, A., Muller, A., Haeni, M., Skinner, C., Fliessbach, A., Buchmann, N., Mäder, P., Stolze, M., Smith, P., Scialabba, N. E.-H., & Niggli, U. (2012). Enhanced top soil carbon stocks under organic farming. Proceedings of the National Academy of Sciences, 109(44), 18226-18231.
- Ghabbour, E. A., Davies, G., Misiewicz, T., Alami, R. A., Askounis, E. M., Cuozzo, N. P., Filice, A. J., Haskell, J. M., Moy, A. K., Roach, A. C., & Shade, J. (2017). National comparison of the total and sequestered organic matter contents of conventional and organic farm soils. Advances in Agronomy, 146, 1-35.
If you want to learn more about Regenerative agriculture, check out Agri AI : Smart Farming Advisor and feel free to ask any questions!
Leave a Reply